PHYSICS OF LASER LIGHT
Visual light is electromagnetic radiation, as are radio waves,
x-rays, and gamma rays. These different classes of electromagnetic radiation are
similar phenomena that occur at very different wavelengths. In 1864, Maxwell[1]
explained that light is an electromagnetic wave with combined electrical and magnetic
oscillations that propagates at 299,792,458 m/sec. Max Planck[2]
subsequently discovered the photoelectric effect; light of a certain color (e.g.,
blue) causes metal to eject electrons at a rate proportional to the brightness of
the light, whereas intense light at other wavelengths (e.g., red, orange, yellow)
cannot. Planck's discovery was the first step toward laser physics, because explaining
the photoelectric effect required a new conceptual framework. Einstein[3]
developed this framework, quantum mechanics, in 1905, establishing the theoretical
basis for laser action. He explained that all electromagnetic radiation (e.g., light,
radio, x-ray) consists of photons, which may be described as follows:
- Their properties are consistent with both particles and waves.
- They propagate in a vacuum, without diminishing, at a constant speed of
299,792,458 m/sec.
- Their energy is proportional to their vibrational frequency:
E (joules) = hv
In this equation, h is the Planck constant, 6.63 × 10-34
(joule-seconds),
and v is the frequency of the photon in Hertz.
- Their wavelength (λ) can be calculated as follows:
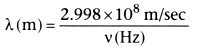
TABLE 67-1 -- Glossary of laser terminology
Ablation: Removal of tissue by
vaporization |
Absorption: The transformation,
by interaction with matter, of radiant energy to a different form of energy |
Active medium: A material that
acts as a laser with the proper excitation |
Aiming beam: A very low-powered
laser beam that is collimated with the high-powered, often invisible therapeutic
beam to illuminate the target site |
Ångstrom unit (Å):
10-10
m |
Anode: The positive terminal
in a gas discharge laser |
Attenuation: The reduction in
beam energy by absorption or scattering as it passes through matter |
Brewster windows: Transparent
windows at the ends of a gas laser discharge tube set at such an angle to the optical
axis of the tube (Brewster angle) as to provide maximum light transmission |
Coherent light: Light in which
the photons all have the same wavelength and maintain a constant in-phase relationship
with each other |
Collimation: The property of
a light beam that describes the degree to which the constituent photons move in a
single direction; highly collimated beams do not spread in diameter as they move
away from the source |
Continuous wave (CW) mode: A
mode of operation in which the laser discharge is continuous |
Diffraction: The modulation in
intensity and apparent bending exhibited by photons as they pass through an opaque
body |
Dopant: A chemical added to a
crystal matrix to serve as an active laser constituent |
Energy density: The amount of
energy per unit area arriving at a surface, usually expressed in joules per square
centimeter |
Excimer: Excited dimer; a type
of laser based on the transition states of a diatomic molecule (e.g., ArF, KrF, XeCl);
these lasers produce photons of very high energy |
Extinction length: The thickness
of a specified medium that absorbs 98% of the incident beam intensity (expressed
as wave number in cm-1
) |
Infrared: Electromagnetic radiation
whose wavelengths lie in the band 0.7 µm to 1.0 mm (i.e., longer than visible
light, but shorter than microwave or radio waves) |
Interference: The phenomenon
in which photons of like wavelength but different phase combine constructively and
destructively to modulate the resulting intensity |
Joule (J): A unit of energy (1
joule = 1 watt-second) |
Micrometer (µm):
10-6
m |
Mode: A description of the intensity
cross section of a laser beam |
Monochromatic light: Light of
a single wavelength or color |
Nanometer (nm): 10-9
m |
Neodymium (Nd): A rare earth
metal, frequently chosen as a laser material within a substrate of glass or yttrium-aluminum-garnet
(YAG) |
Output power: Rate of energy
discharge, usually measured in watts or joules per second |
Photon: A quantum of electromagnetic
energy possessing both wavelike and particle-like properties; photons travel at a
constant speed of approximately 300,000,000 m/sec |
Power density: The amount of
power (energy per second) per unit area arriving at a surface, usually expressed
in watts per square centimeter |
Pulsed mode: A mode of operation
in which the laser delivers discrete (usually quite brief) bursts of photons |
Pump: The means of including
an electron population inversion so that stimulated emission may occur |
Resonator: The combination of
laser material and mirrors necessary to support laser activity |
Spontaneous emission: The emission
of a photon when an excited orbital electron decays back to its ground-state energy |
Stimulated absorption: The process
by which an orbital electron captures the energy of a colliding photon and is boosted
to higher energy orbital |
Stimulated emission: The process
by which an electron in a high-energy orbital, if struck by an appropriate photon,
emits a new photon of wavelength, phase, and direction equal to that of the original,
colliding photon |
Tunable laser: A laser that can
be adjusted so as to provide a selected output wavelength from a range of possibilities |
Ultraviolet: Electromagnetic
radiation having wavelengths shorter than visible light, in the range between 0.01
and 0.38 µm |
Wavelength: The distance from
peak to peak of a photon wave; the usual units for light waves are nanometers (nm)
or micrometers (µm) |
YAG: A synthetic crystalline
matrix composed of yttrium, aluminum, and garnet, with the chemical formula Y3
Al5
O12
|
Wavelengths of visible light range from 385 to 760 nm (nanometer:
10-9
m); shorter wavelengths are ultraviolet, and longer wavelengths
are infrared. Einstein also explained that the photoelectric effect is independent
of the number of photons present, occurring even if only one photon at a time struck
the metal surface. Frequency is the key; only photons of high enough energy (i.e.,
high enough frequency) can provide the energy necessary to stimulate electron emission;
lower-energy photons, even arriving in large numbers at a given time (i.e., brightness),
cannot. These findings precipitated the development of laser light.
Some additional aspects of quantum physics provide greater insight
into the origin and nature of laser light. For example, electrons circling the nuclei
of atoms are constrained to a few specific orbital patterns and radii ( Fig.
67-1
). Each orbital is associated with a specific energy level; the only
way an electron can move from one orbital
Figure 67-1
Electronic orbitals and energy levels are shown in this
schematic diagram of the Bohr model of the hydrogen atom. The circular electron
orbitals are a simplification of the probabilistic electron cloud structure. Superimposed
on the electron orbitals are the energy transitions responsible for two of the line
series in the emission spectrogram of hydrogen. The Lyman series of spectral lines
occurs when electrons release photons as they jump down to the lowest-energy orbital;
the Balmer series of lines represents jumps to an orbital just above the lowest.
The wavelength of the resulting photons is inversely proportional to the size of
the jump. For example, in the Balmer series, the jump from the n = 3 orbital to
the n = 2 orbital releases a photon of wavelength 656.3 nm, whereas the jump from
n = 5 to n = 2 is more energetic, releasing a 434-nm photon.
to another of higher or lower energy is to absorb or emit, respectively, an amount
of energy exactly equal to the difference in energy between the two orbitals. The
process by which a photon's energy is captured by an electron, thereby vaulting the
electron into a higher energy orbital, is called stimulated
absorption. ( Fig. 67-2
);
the converse process by which an electron drops to lower orbital, in the process
sending out a photon to carry away the excess energy, is called spontaneous
emission. In 1917, Einstein predicted that stimulated
emission, the key to laser action,[4]
also could occur. For this process, a photon of a particular wavelength (i.e., energy)
must collide with an atom ready for spontaneous emission at that wavelength (i.e.,
having an electron already in the higher energy orbital), stimulating immediate photon
emission and electron descent to a lower orbital. The colliding photon leaves the
electron with the emitted photon, with both photons having the identical wavelength,
phase, and direction.
The energy differences between electron orbitals are specific
to different atoms and are responsible for the fingerprint-like emission (i.e., absorption
spectra) used in chemical identification. Under normal circumstances of thermodynamic
equilibrium, most of a substance's electrons are in the lowest available (ground-state)
energy orbitals. Normally, an electron is much more likely to engage in spontaneous
than in stimulated emission (by a factor of 1033
:1). The key to the creation
of the laser was the achievement of a population inversion,
in which many
Figure 67-2
Absorption, emission, and stimulated emission. Photons
may interact with orbital electrons in three ways. A,
A photon striking an electron may transfer its energy to the electron, pushing the
electron into a higher-energy orbit. This interaction is known as absorption.
B, An electron in an orbit higher than the ground
(i.e., minimal energy) state may spontaneously lose energy in the form of an emitted
photon. C, An incoming photon may interact with an
electron that is already in a high-energy orbit, with the result that two perfectly
coherent, collimated photons leave the electron; this is known as stimulated
emission.
electrons are "pumped up" to the higher orbital, waiting for a photon to come along
and start a chain reaction (i.e., amplification) of stimulated emission. In 1958,
the technology for pumping up electrons to achieve stimulated emission was introduced.
[4]
A method was found for enclosing the laser
medium
between parallel mirrors, so that laser-frequency photons could traverse the medium
repeatedly and maximize the number of stimulated emissions. When one of the mirrors
is partly transparent, a beam of laser light eventually exits through that mirror.
Laser light differs from ordinary light in three ways. First,
it is highly monochromatic. It consists of photons that have a well-defined, very
narrow band of wavelengths, whereas ordinary light contains a wide spectrum of wavelengths.
Second, laser light is coherent, a property that implies that the electromagnetic
fields of all photons in the laser beam oscillate synchronously in identical phase.
In ordinary light, the electromagnetic fields are phased randomly even at the same
wavelength. Third, directed beams of laser light are collimated (i.e., have minimal
dispersion). The light remains in a narrow, collimated beam, whereas ordinary light
beams spread out in all directions from a point source. These three characteristics
allow lasers to generate intense light beams, to send such beams efficiently and
accurately through lenses, and to deliver intense energy to small target sites.