Structure-Activity Relationships and Physicochemical
Properties
The intrinsic potency and duration of action of local anesthetics
are clearly dependent on certain features of the molecule.
Lipophilic-Hydrophilic Balance
The lipophilic versus hydrophilic character of local anesthetics
depends on the size of the alkyl substituents both on or near the tertiary amine
and on the aromatic ring. "Lipophilicity" expresses the tendency of a compound to
associate with membrane lipids, which is usually approximated by equilibrium partitioning
into a hydrophobic solvent such as octanol.[1]
Such octanol/buffer partition coefficients are comparable to membrane/buffer partition
coefficients for the uncharged species of local anesthetics but greatly underestimate
membrane partitioning for the charged, protonated species, octanol being a poor model
for the polar regions near the membrane surface.[2]
In this chapter we use the term hydrophobicity,
expressed by octanol/buffer partitioning, to describe a physicochemical property
of local anesthetics.
Compounds with a more hydrophobic nature are obtained by increasing
the size of the alkyl substituent or substituents. These agents are more potent
and produce longer-lasting blocks than their less hydrophobic congeners do.[3]
[4]
[5]
For example,
etidocaine, which has three more carbon atoms than lidocaine has in the amine end
of the molecule, is four times as potent and five times as long lasting when compared
for impulse blockade in the isolated sciatic nerve.
Hydrogen Ion Concentration
Local anesthetics in solution exist in a rapid chemical equilibrium
between the basic uncharged form (B) and the charged cationic form (BH+
).
At a certain hydrogen ion concentration (log10
-1
[-pH]) specific
for each drug, the concentration of local anesthetic base in solution is equal to
the concentration of charged cation. This hydrogen ion concentration is called pKa
.
The relationship is defined by

pKa
values for standard
local anesthetics are listed in Table
14-2
. The tendency to be protonated also depends on environmental factors,
such as temperature and ionic strength, and on the medium surrounding the drug.
In the relatively apolar milieu of a membrane, the average pKa
of local anesthetics is lower than in solution.[6]
This is chemically equivalent to saying that the membrane concentrates the base
form of the local anesthetic more than it concentrates the protonated cation form.
The pH of the medium containing the local anesthetic influences
drug activity by altering the relative percentage of the basic or protonated forms.
For example, in inflamed tissue, the pH is lower than normal, and local anesthetics
are more protonated than in normal tissue and thus penetrate the tissue relatively
poorly (see later).
The relationship between pKa
and the percentage of local anesthetic present in the cationic form is shown in
TABLE 14-1 -- Representative local anesthetics in common clinical use
Generic
*
and Common Proprietary Name |
Chemical Structure |
Approximate Year of Initial
Clinical Use |
Main Anesthetic Use |
Representative Commercial Preparation |
Cocaine |
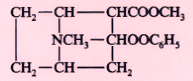
|
1884 |
Topical |
Bulk powder |
Benzocaine (Americaine) |
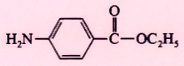
|
1900 |
Topical |
20% ointment |
|
|
|
Topical |
20% aerosol |
Procaine (Novocain) |
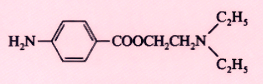
|
1905 |
Infiltration |
10- and 20-mg/mL solutions |
|
|
|
Spinal |
100-mg/mL solution |
Dibucaine (Nupercaine) |
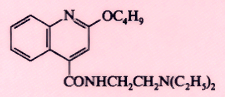
|
1929 |
Spinal |
0.667-, 2.5-, and 5-mg/mL solutions |
Tetracaine (Pontocaine) |
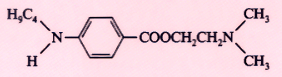
|
1930 |
Spinal |
Niphanoid crystals—20- and 10-mg/mL solutions |
Lidocaine (Xylocaine) |
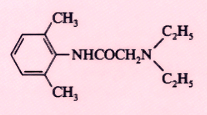
|
1944 |
Infiltration |
5- and 10-mg/mL solutions |
|
|
|
Peripheral nerve blockade |
10-, 15-, and 20-mg/mL solutions |
|
|
|
Epidural |
10-, 15-, and 20-mg/mL solutions |
|
|
|
Spinal |
50-mg/mL solution |
|
|
|
Topical |
2.0% jelly, viscous |
|
|
|
Topical |
2.5%, 5.0% ointment |
Chloroprocaine (Nesacaine) |
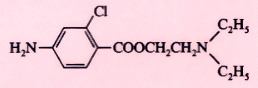
|
1955 |
Infiltration |
10-mg/mL solution |
|
|
|
Peripheral nerve blockade |
10- and 20-mg/mL solutions |
|
|
|
Epidural |
20- and 30-mg/mL solutions |
Mepivacaine (Carbocaine) |
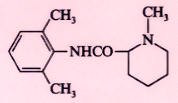
|
1957 |
Infiltration |
10-mg/mL solution |
|
|
|
Peripheral nerve blockade |
10- and 20-mg/mL solutions |
|
|
|
Epidural |
10-, 15-, and 20-mg/mL solutions |
Prilocaine (Citanest) |
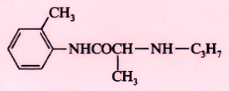
|
1960 |
Infiltration |
10- and 20-mg/mL solutions |
|
|
|
Peripheral nerve blockade |
10-, 20-, and 30-mg/mL solutions |
|
|
|
Epidural |
10-, 20-, and 30-mg/mL solutions |
Bupivacaine (Marcaine) |
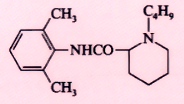
|
1963 |
Infiltration |
2.5-mg/mL solution |
|
|
|
Peripheral nerve blockade |
2.5- and 5-mg/mL solutions |
|
|
|
Epidural |
2.5-, 5-, and 7.5-mg/mL solutions |
|
|
|
Spinal |
5- and 7.5-mg/mL solutions |
Ropivacaine (Naropin) |
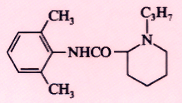
|
1992 |
Infiltration |
2.5- and 5-mg/mL solutions |
|
|
|
Peripheral nerve blockade |
5- and 10-mg/mL solutions |
|
|
|
Epidural |
5- and 7.5-mg/mL solutions |
Modified from Covino B, Vassallo H: Local Anesthetics:
Mechanisms of Action and Clinical Use. Orlando, FL, Grune & Stratton, 1976. |
*USP
nomenclature
TABLE 14-2 -- Relative in vitro conduction-blocking potency and physicochemical properties
of local anesthetics
|
|
Physicochemical Properties |
Drug |
Relative Conduction-Blocking
Potency
*
|
pKa
†
|
Hydrophobicity
†
|
Low-potency procaine |
1 |
8.9 |
100 |
Intermediate potency |
|
|
|
Mepivacaine |
1.5 |
7.7 |
130 |
Prilocaine |
1.8 |
8.0
‡
|
129 |
Chloroprocaine |
3 |
9.1 |
810 |
Lidocaine |
2 |
7.8 |
366 |
High potency |
|
|
|
Tetracaine |
8 |
8.4 |
5822 |
Bupivacaine |
8 |
8.1 |
3420 |
Etidocaine |
8 |
7.9 |
7320 |
From Strichartz GR, Sanchez V, Arthur GR, et al:
Fundamental properties of local anesthetics. II. Measured octanol:buffer partition
coefficients and pKa values of clinically used drugs.
Anesth Analg 71:158–170, 1990. |
*Data derived from
C fibers of isolated rabbit vagus and sciatic nerve.
†pKa
and hydrophobicity at 36°C; hydrophobicity equals the octanol buffer partition
coefficient of the base. Values are ratios of concentrations.
‡Values at
25°C.
Figure 14-2
. As described
later, pH has dual effects on clinical effectiveness, depending on the location at
which the local anesthetic is injected and the importance of the base form for tissue
penetration.